Learn
Blood Spatter
Before we go any further let’s make sure everyone understands that the correct terminology is blood spatter NOT blood splatter. Many people make this mistake. Blood is a fluid and follows the law of physics. Blood spatter analysis focuses on the physical properties of blood and the patterns that blood produces when different forces are applied to the blood. Blood spatter analysis helps crime scene investigators figure out what happened at a crime scene. From examining the blood spatter, forensic scientists can determine many things. Here are a few examples:
- Distance between the origin of blood and the impact area (bloodstain)
- Where the blood came from
- Direction of an object or person
- Number of shots or blows to victim
- Type of weapon used
- If the assailant was right- or left-handed
- Type of impact (low-, medium- or high-velocity) and direction of impact
- If the victim died immediately or later
- Where the victim was during bloodshed
- If the victim or other objects were moved after bloodshed
From these, forensic scientists develop a reconstruction theory of what happened before, during, and after the crime.
Let’s first look at how blood drops are analyzed and some terminology you will use throughout this unit. The first, main blood droplet that strikes a surface is known as the parent drop. From the parent drop, small drops can break off and create satellite spatters. These satellite spatters can tell blood analysts what direction the blood is traveling. When a blood droplet strikes a surface, spines can radiate out from the parent drop and tell what direction the blood is traveling.
Blood Droplets
Even though many people think of a blood droplet as a teardrop shape, it has more of a spherical shape. This is due to the surface tension that binds the molecule together. This property makes blood have the tendency to contract. This spherical shape is kept in space until it hits a surface. A bloodstain is then formed.
Research has shown that blood droplets falling on similar surfaces at the same height will produce the same basic shape. As the height increases, the diameter of the bloodstain produced by a droplet also increases.
Once the blood reaches a certain height, it reaches what is called terminal velocity. This height is about 2.2 meters. Above this height the diameter doesn’t change much. When a droplet falls from 90 degrees (pop-up or perpendicular to the ground), generally a round-shaped bloodstain will form.
The shape of the bloodstain is affected by many factors. These include:
- Height (discussed above)
- Size of the droplet
- The angle from which the blood came
- Velocity from the origin of blood
- Texture of surface
- Glass- smooth outside edges
- Rough surface-scalloping edges with spines
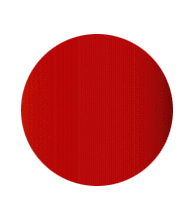
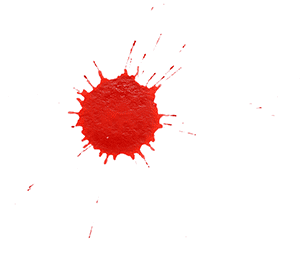
Droplet Flight Dynamics
Blood exposed from an open wound, or present in sufficient volume on an object, such as this knife, will be pulled to the lowest point on it source surface.
As the droplet increase in volume, the forces of gravity will eventually overcome the combination of the droplet’s surface tension and its adhesive force to the object, and will pull the droplet away from the object’s surface and towards the ground. This drop can also be affected by
inertial forces as the weapon is swung, but in the simplest
scenario, gravity and surface tension play the greatest role.
As gravity causes more and more blood to flow to the collection point, the opposing forces of surface tension and gravity will cause the blood to be retained in what is called a passive drop. Eventually, enough blood will have flowed into the passive drop that its weight will allow gravity to overcome the surface tension and pull the drop away from the object.
As the blood droplet pulls away from the object, the surface tension of the droplet will cause the teardrop shape that it forms to retract back in towards the center of the droplet. This ‘snap-back” forces starts the droplet oscillating between spheroid and spheroid-oblong. As the droplet’s surface tension evens out across its entire surface, the oscillation decays, and the drop takes on a perfectly spherical shape.
As the droplet falls, it again encounters two opposing forces (assuming, in this example, that is falls straight down). One is gravity, which accelerates the droplet and imparts to it force, based on its mass. The other is friction that the droplet encounters with the air, in direct proportion to is speed. Once gravity has brought the droplet to a speed at which the force of the air’s friction is equal to the downward force of gravity, the droplet will no loner accelerate, but will maintain this speed, known as its terminal velocity.
A larger droplet experiences the same acceleration due to gravity, but due to is great mass, this translates into a greater downward force (F = ma). Although the larger droplet does have a greater surface area than the smaller droplet, and therefore experiences more friction from the air, the volume has increased to an even greater scale. Recall, volume is a 3rd degree formula, while surface area is only a 2nd. Therefore, even with its greater surface area, the larger droplet is still able to reach a higher speed than the smaller one before developing sufficient friction to counteract its downward force; its terminal velocity is higher.
Four Phases
Regardless of the surface onto which a blood droplet is falling, the angle or velocity at which it does so, or the volume of the droplet, there are four distinct phases involved in the reaction of a moving droplet with impact against a surface. Let’s look at those phases.
Phase 1: Contact and Collapse. Upon initial contact with the surface, the droplet begins to collapse, from the bottom upward. The collapse occurs at the portion of the droplet currently contacting the surface, while the portions above remain intact. As this collapse progresses, the displaced blood is pushed outward. In this case, it forms an outwardly-advancing rim around the collapsing droplet. At acute angles, the rim forms on the side of the droplet opposite the angle of impact, and is pushed in that direction.
Phase 2: Displacement. Once the droplet has finished collapsing, most of the blood that has not yet been pushed into the rim is carried there by its remaining outward inertia. Slight dimples and spines begin to form on the edge of the pool, based on irregularities on the impact surface, but so far, the surface tension of the blood has not bee broken, and it still forms essentially a single mass, despite having undergone considerable change in shape.
Phrase 3: Dispersion. Blood is forced into the boundary rim and into protrusions that rise upward. As the volume of blood in the rim and protrusions becomes unstable, inertia begins to separate the structures, resulting in the creation of satellite spatter. In the case of a droplet hitting a surface at 90 degree, this takes the form of a crown or blossom effect. At more acute angles, a wave cast-off occurs on the side of the rim farthest from the angle of impact.
Phase 4: Retraction. Recall that as the force of the droplet’s impact on the surface is trying to throw the mass of blood apart, the surface tension is still trying to hold it together as a single unit. In this phase the surface tension finally overcomes the blood’s waning momentum. The spines between the satellite spatters and the parent stain narrow as blood is drawn into one or the other. Satellite spatters that have enough momentum are able to break off complete in the phase, forming separate stains.